ONH Biomechanics and Glaucoma: A Complicated Story

by J. Crawford Downs
Why ONH and peripapillary scleral biomechanics are key factors in glaucoma
The ONH is of particular interest from a biomechanical perspective because it is a weak spot within an otherwise strong corneo-scleral envelope. The preponderance of evidence suggests that the lamina cribrosa is the principal site of retinal ganglion cell (RGC) axonal insult in glaucoma. In this sense, glaucomatous optic neuropathy can be viewed as an axonopathy, where damage to the visual pathway is driven by insult to RGC axons as they exit the eye at the ONH. Hence, neither neuroprotection of the RGC soma or neuroregeneration of the RGC axons is likely to be effective in preventing, slowing or reversing vision loss in glaucoma unless the pathologic environment in the ONH is also simultaneously addressed. As such, clinical glaucoma management is a three-legged stool in which the health of the RGC soma, its axon, and the axonal pathway to the brain must be simultaneously supported and maintained to prevent vision loss. The mechanisms of RGC axonal insult at the ONH are poorly understood, but we present a framework of IOP-driven ONH biomechanics as a central mechanism in the pathophysiology of glaucoma.
The lamina cribrosa provides structural and functional support to the RGC axons as they pass from the relatively high-pressure environment in the eye to a low-pressure region in the retrobulbar cerebrospinal space. To protect the RGCs in this unique environment, the lamina cribrosa in higher primates developed as a complex structure composed of a three-dimensional network of flexible beams of connective tissue (Fig. 1). The ONH is nourished by the short posterior ciliary arteries, which penetrate the immediate peripapillary sclera to feed capillaries contained within the laminar beams. This intra-scleral and intra-laminar vasculature is unique in that it is encased in load-bearing connective tissue, either within the scleral wall adjacent to the lamina cribrosa, or within the laminar beams themselves. Glaucoma is a multifactorial disease, and we believe that biomechanics not only determines the mechanical environment in the ONH, but also mediates cellular responses through mechanotransduction and IOP-related reductions in blood flow and through various pathways (Fig. 2). Consideration of the anatomy of the lamina cribrosa and peripapillary sclera alone suggests that the classic 'mechanical' and 'vascular' mechanisms of glaucomatous injury are inseparably intertwined.
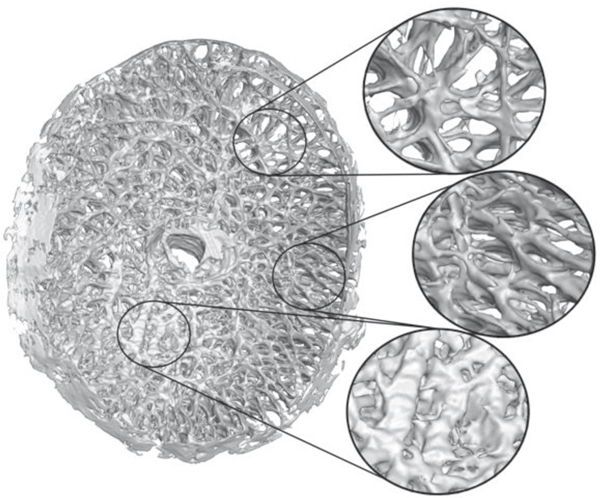
Fig. 1. High-resolution 3D reconstruction of the lamina cribrosa. Note the regional variation in both connective tissue density and predominant laminar beam orientation throughout the laminar structure. These spatial variations in micro-architecture will affect the regional deformation and load-bearing characteristics of the lamina cribrosa both as a whole and at the level of the individual laminar beams. (Adapted from 3.)
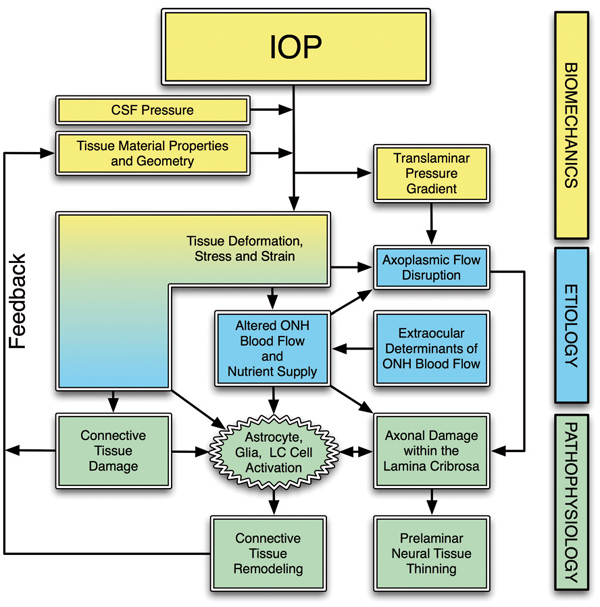
Fig. 2. The biomechanical paradigm of glaucoma. IOP acts mechanically on the ocular tissues, producing deformations, strain and stress. Local tissue deformations depend on the geometry and material properties of an individual eye. In a biomechanical paradigm, stress and strain alters the blood flow (primarily), and the delivery of nutrients (secondarily) through remodeling-driven alterations in connective tissue stiffness and diffusion properties. IOP-related stress and strain also cause connective tissue damage directly, or indirectly through cellular mechanisms, which induces connective tissue remodeling that alters the ONH's response to loading via a feedback mechanism. These effects are pertinent at all time scales, and combine to cause axonal damage in the laminar region. (Adapted from 3.)
To incorporate these concepts into an overarching conceptual framework, we have proposed that the ONH is a biomechanical structure (Fig. 2). This paradigm assumes that IOP-related stress (force distribution) and strain (local relative deformation) are central determinants of both the physiology and pathophysiology of the ONH tissues at all levels of IOP. IOP-induced changes to the ONH include alterations to the load-bearing connective tissues of the lamina cribrosa and the peripapillary sclera, as well as blood flow alterations and changes in the cellular components.
The biomechanics of the ONH and peripapillary sclera are very complex (Fig. 4). IOP-related stress generates strain patterns in the ONH and peripapillary sclera that are not only dependent on local connective tissue geometries (Fig. 1) and material properties, but are also influenced by complex dynamic loading conditions. The important factors governing the ONH's response to IOP include the alignment and density of collagen fibrils in each tissue (stiffness and anisotropy), the regional density and thickness of the tissue, the rate of change in IOP (via tissue viscoelasticity) and the level of IOP-related strain at the time of altered loading (via tissue nonlinearity). In broad terms, the ONH connective tissues should be stiffer when there is already considerable strain present and/or if the IOP load is applied quickly. Conversely, the ONH should be more compliant in response to slow changes in IOP and/or at low levels of strain. In addition, studies have shown that mechanical strain is higher in regions of ONH where laminar density is lower. It is important to note that the ONH responds to IOP elevations as a structural system, so the acute mechanical response of the lamina cribrosa is confounded with the responses of the peripapillary sclera, prelaminar neural tissues, and retrolaminar optic nerve. Also, because the lamina cribrosa lies underneath the prelaminar neural tissues and the acute structural responses of these two tissues to acute IOP elevations are quite different, acute laminar deformation cannot be directly measured from imaging the surface topography of the ONH. A final confounding effect is the cerebrospinal fluid pressure, which along with IOP determines the translaminar pressure gradient that must be borne by the lamina cribrosa.
Intuitively, it may seem that for a given acute increase in IOP, the lamina cribrosa should deform posteriorly. Recent histologic and invivo OCT imaging studies have shown that the lamina cribrosa does not consistently deform posteriorly when IOP increases. In some eyes, the lamina moves anteriorly with respect to Bruch's membrane opening. Thus, our current understanding of the aggregate response of the ONH to acute IOP elevation is that expansion of the scleral canal pulls the lamina taut within the plane of the sclera to a variable degree, making it more resistant to posterior deformation out of that plane (Fig. 3). It is important to note that the apparent lack of anterior-to-posterior laminar deformation with acute IOP elevation does not mean that the lamina is not strained. In this scenario, the expansion of the canal stretches the lamina cribrosa within the plane of the sclera generating substantial strain within the laminar beams.
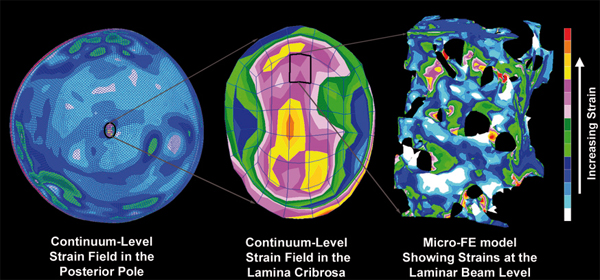
Fig. 3. Complexities in the laminar biomechanical response. Left: the strain distribution in a macro-scale model of the connective tissues of the posterior pole of the eye. Note that thickness variations in the sclera give rise to a non-uniform distribution of strain within the shell and that the strains are lower in the sclera than in the more compliant lamina cribrosa. Middle: detail of the strain field within the macro-scale representation of the lamina cribrosa. While this portion of the model has been assigned regional material properties related to the amount and orientation of the laminar beams (based on 3D reconstruction data such as in Fig. 1), the continuum description represents a bulk homogenization of the specific micro-architecture in each element. Right: the distribution of mechanical strain at the micro-scale in the laminar beam micro-architecture. (Adapted from 3.)
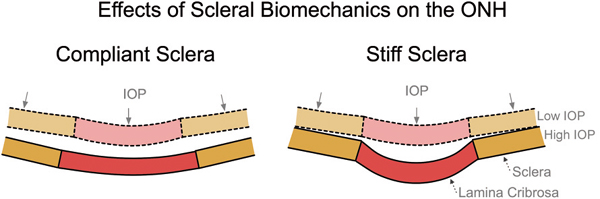
Fig. 4. The influence of scleral mechanics on ONH mechanics. IOP induces large scleral canal expansions in eyes with compliant sclera (left) that pulls the contained lamina taut despite the direct posterior force of IOP on the laminar surface. Conversely, a stiff sclera allows relatively little canal expansion with IOP elevation (right) and less stretching of the contained lamina, thus allowing the lamina to be displaced posteriorly by the direct action of IOP on its anterior surface. (Adapted from 3.)
The IOP-glaucoma relationship
Although clinical IOP-lowering remains the only proven method of preventing the onset and progression of glaucoma, the role of IOP in the development and progression of the disease remains controversial. This largely arises from the clinical observation that significant numbers of patients with normal IOPs develop glaucoma (e.g., normotensive glaucoma), while other individuals with elevated IOP show no signs of the disease. This could mean that IOP (or some factor driven by IOP) is a primary causative factor in glaucoma, and susceptibility to IOP varies between individuals. Another possibility is that clinical characterization of mean IOP using infrequent snapshot measurements fails to capture exposure to larger, injurious IOP fluctuations that are partly driving the disease in these 'normotensive' glaucoma patients, which undermines the study of the IOP-glaucoma relationship. IOP telemetry data in both humans and animal models also show that IOP is extremely variable from second-to-second (Fig. 5), minute-to-minute, hour-to-hour, and day-to-day, which suggests that snapshot clinical measurements of IOP give the clinician only limited understanding of the true IOP to which a patient is exposed. Very little is known about IOP fluctuations in humans and how the eye responds to those fluctuations, but IOP levels at all timescales have the potential to injure the RGC axons in the ONH. Recent data from IOP telemetry in non-human primates suggests that up to 10% of the total IOP mechanical energy that the must absorb during waking hours is due to the short-term spikes in IOP from resulting from blinks, saccades, squints and other external traction and pressure forces on the eye. It may be that one of the underlying reasons that many studies do not demonstrate a more direct relationship between IOP and glaucomatous onset and progression, is that we don't know what IOP truly is in the patients we study, and/or we are ignoring injurious short-term IOP fluctuations entirely.
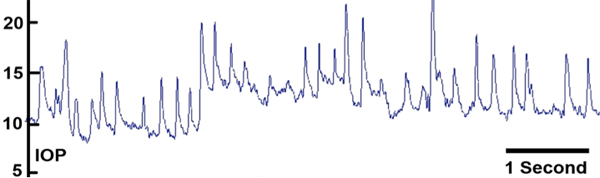
Fig. 5. IOP dynamics. Screen capture of approximately seven seconds of the continuous IOP telemetry signal in an awake, unrestrained non-human primate showing the dynamic nature of IOP, showing the IOP fluctuations resulting from blinks and saccades. (Adapted from 5.)
Whether it is mean IOP and/or IOP fluctuations that drive glaucomatous pathogenesis, there is a wide spectrum of individual susceptibility to IOP-related glaucomatous vision loss. The biomechanical effects of IOP on the tissues of the ONH likely play a central role in the development and progression of the disease at all IOPs and on all time scales, from slow nocturnal variations to short-term IOP spikes from saccades and blinks. The individual susceptibility of a particular patient's ONH to IOP insult is likely a function of the biomechanical response of the constituent tissues and the resulting mechanical, ischemic and cellular events driven by that response. Hence eyes with a particular combination of tissue geometry and material properties may be susceptible to damage at normal IOP, while others may have a combination of ONH tissue geometry and material properties that can withstand even high levels of IOP and IOP fluctuation.
Aging, biomechanics, and glaucoma
Glaucoma is primarily a disease of aging, and recent work has shown that the sclera and lamina cribrosa stiffen significantly with age. One might assume this to be protective against mechanical strain, in that stiffer connective tissues resist mechanical strain better than more compliant tissues. It is important to note however that this stiffening also induces larger IOP spikes related to blinks, saccades, and vascular filling, in that the eye is less able to elastically expand to absorb IOP energy. Hence, it may be that in the process of age-related connective tissue stiffening, the eye has remodeled to a state that is actually more susceptible to IOP spikes. Recent studies have also suggested that age-induced vascular deficiencies, as well as age-related loss of metabolic efficiency are also likely to contribute to the increased risk of glaucoma in the elderly.
How might we assess biomechanical risk to the ONH clinically?
There are currently no science-based tools to predict at what level of IOP an individual ONH will be damaged. Eventually, knowing the relationship between IOP, IOP fluctuations, mechanical strain- or stress-driven remodeling, ONH blood flow, and astrocyte and axonal homeostasis will drive the clinical assessment of safe target IOP, although the technologies to assess these factors have yet to materialize. Clinical characterization of the actual IOP insult through continuous, telemetric IOP monitoring will eventually allow us to understand the biomechanical loads in the eye, a critical component to understanding the forces driving glaucoma pathophysiology. Finally, targets for deep (sub-surface) ONH imaging will likely allow early detection of glaucomatous remodeling and damage to the lamina cribrosa. Once clinically detectable, early stabilization and perhaps reversal of these pathophysiological changes will become new patient-specific endpoints to determine a safe target IOP.
References
- Burgoyne CF, Downs JC, Bellezza AJ, Hart RT. The optic nerve head as a biomechanical structure: a new paradigm for understanding the role of IOPrelated stress and strain in the pathophysiology of glaucomatous optic nerve head damage. Progr Ret Eye Res 2005; 24: 39-73.
- Downs JC, Roberts MD, Burgoyne CF. Mechanical Environment of the Optic Nerve Head in Glaucoma. Optom Vis Sci 2008; 85: 425-43
- Sigal IA, Roberts MD, Girard M, Burgoyne CF, Downs JC. Biomechanical Changes of the Optic Disk. In: Levin LA, Albert DM (Eds.), Ocular Disease: Mechanisms and Management, 1st ed. London: Elsevier, 2010.
- Downs JC, Roberts MD, Sigal IA. Glaucomatous cupping of the lamina cribrosa: A review of the evidence for active progressive remodeling as a mechanism. Exper Eye Res 2011; 93: 133-140.
- Downs JC, Burgoyne CF, Seigfreid WP, Reynaud JF, Strouthidis NG, Sallee V. 24-hour IOP Telemetry in the Non-human Primate: Implant System Performance and Initial Characterization of IOP At Multiple Timescales. Invest Ophthalmol Vis Sci 2011; 52(10): 7365-7375.